Large dams trap a great deal of river sediment. But in many cases this does not result in a significant reduction in sediment delivery by rivers to the coast. This is due largely to the fact that the lower reaches of many coastal plain rivers were sediment bottlenecks long before the dams were built, and did not deliver much sediment to the coast to start with, and to the long under-appreciated importance of sediment sources in the lower coastal plain and within the coastal zone.
This has been known, at least in some case studies, for 30 years. However, these case studies have done little to offset the conventional wisdom that because (A) dams trap sediment (100 percent of bedload and often >90 percent of suspended load), and (B) rivers are an important source of coastal sediments, then (C) sediment delivery to the coast has been reduced to the coastal zone since a proliferation of dam-building in the 1950s and 1960s, leading to problems such as beach erosion and wetland loss.
The recent publication of Coastal sedimentation across North America doubled in the 20th century despite river dams inspired me to revisit my own work on this subject. And this post is indeed mainly a review of my own experiences, rather than a comprehensive review of the topic. The abstract of the article, published in 2020 by Antonio Rodriguez, Brent McKee and colleagues, reads thusly:
The proliferation of dams since 1950 promoted sediment deposition in reservoirs, which is thought to be starving the coast of sediment and decreasing the resilience of communities to storms and sea-level rise. Diminished river loads measured upstream from the coast, however, should not be assumed to propagate seaward. Here, we show that century-long records of sediment mass accumulation rates (g cm−2 yr−1) and sediment accumulation rates (cm yr−1) more than doubled after 1950 in coastal depocenters around North America. Sediment sources downstream of dams compensate for the river-sediment lost to impoundments. Sediment is accumulating in coastal depocenters at a rate that matches or exceeds relative sea-level rise, apart from rapidly subsiding Texas and Louisiana where water depths are increasing and intertidal areas are disappearing. Assuming no feedbacks, accelerating global sea-level rise will eventually surpass current sediment accumulation rates, underscoring the need for including coastal-sediment management in habitat-restoration projects.
* * * * *
First, let me reiterate than points (A) and (B) above are true and accurate. And, in some cases—mainly relative short, steepland rivers with dams close to the coast—(C) is true as well. In addition, we also know that human activities—in North America, particularly since extensive European settlement and expansion—has increased erosion rates. But documented increases in erosion and fluvial sediment loads inland in many cases were not reflected in increased sediment delivery to river mouths.
In many cases this is largely due to extensive colluvial and alluvial sediment storage. Beginning in the 1970s a series of studies demonstrated that rivers are not sediment conveyor belts from inland to ocean, and that large amounts of eroded sediment are stored within drainage basins for years (or longer). I began working on this in the late 1980s in rivers of the North Carolina piedmont, long known as a post-European settlement and 20th century erosional hotspot. In Phillips (1991a) sediment budgets for four large ( > 1000 km2) drainage basins in the Piedmont were estimated from data compiled during erosion and sedimentation surveys. Budgets for the upper Tar, upper Neuse, Haw and Deep River basins showed broadly similar trends in allocation of eroded sediment among yield and storage. Sediment yield as a percentage of mean annual gross erosion within the basins averaged 10%. This was less than the rate of alluvial storage, which averaged 14% of annual gross erosion. About 76% of the mean annual erosion was stored as colluvium on hillslopes. In these basins those trends predated construction of large dams. This was not necessarily the case for a study of the Pee Dee River basin in North and South Carolina (Phillips, 1991b). There, only about four percent of eroded sediment (on an average annual basis) was delivered to the Winyah Bay (SC) estuary. More than two thirds was stored as colluvium before reaching streams, and more than twice as much was trapped in reservoirs as delivered to the bay. But more than twice as much sediment was stored as channel and floodplain alluvium as was sequestered behind dams. A key idea of that study was that (like many rivers that flow across the coastal plain), the Pee Dee is transport-limited. I estimated that an 88 percent reduction in erosion rates would be necessary to result in a noticeable decrease in sediment supply to the estuary. In a third paper (Phillips, 1991c) I linked these concepts to coastal water quality protection. There was, and is, a tendency to blame upstream cities for pollution in N.C. estuaries, but those pollution problems cannot be solved without also dealing with pollution sources near and within the coastal zone!
I took a closer look at the lower Neuse River in Phillips (1992), using a mineralogical tracer of a Piedmont sediment source. This indicated a very small portion of upper-basin sediment reaching the lower Neuse (with limited dam effects!), and no detectable Piedmont signature in the lower 50 km or so of river above the Neuse estuary. Rather, lower basin alluvium has a dominantly coastal plain source. In Phillips (1992b) this general trend was also detected for other large rivers of the region. Reconstructions of pre- and post-colonial sediment dynamics in the lower Neuse River basin indicated massive post-European increases in erosion and stream loads, but with most of the increased sediment production stored as colluvium and alluvium (Phillips, 1993).
* * * * *
In the coastal plain, low relief, gentle slopes, and sandy soils with rapid infiltration had long been thought to be associated with very limited erosion. This impression was probably also promoted by visual comparison with the adjacent piedmont, where exposed subsoils (red clay is an iconic symbol of the southern piedmont) and extensive gullies were common. By this point, the evidence from the river systems called this conventional wisdom into question. So I (and others) began to focus on erosion within the coastal plain. The bottom line is that coastal plain erosion rates are significant, and sometimes in the same ballpark as historical piedmont erosion rates (Phillips, 1993; 1997; Phillips et al., 1993; 1999a;b; Slattery et al., 1998). Again, I am only mentioning work I was involved in—others were coming up with similar findings.
Why? The region also includes clayey soils that readily produce overland flow. And even in the sandy soils, high water tables promote saturation-excess runoff. On agricultural (and some silvicultural) land, artificial drainage systems are specifically designed to prevent ponding and keep surface runoff moving. Further, when runoff does occur the sandy topsoils are readily eroded and the clayey ones often include high contents of kandic clays that are easily dispersed in water (Phillips, 1997; Slattery et al., 1998; Slattery et al., 2006).
* * * * *
In the early 2000s, along with Mike Slattery of Texas Christian University and some of our students, I turned my attention to rivers of the Gulf of Mexico coastal plain in Texas (big shoutout to Greg Malstaff, then with the Texas Water Development Board who was vital in supporting this work). There the rivers feature some very large dams and reservoirs, and the coast has experienced extensive erosion. Many assumed—logically enough, given what was known at the time—a connection between sediment trapping by dams, reduced sediment supply downstream, and coastal erosion.
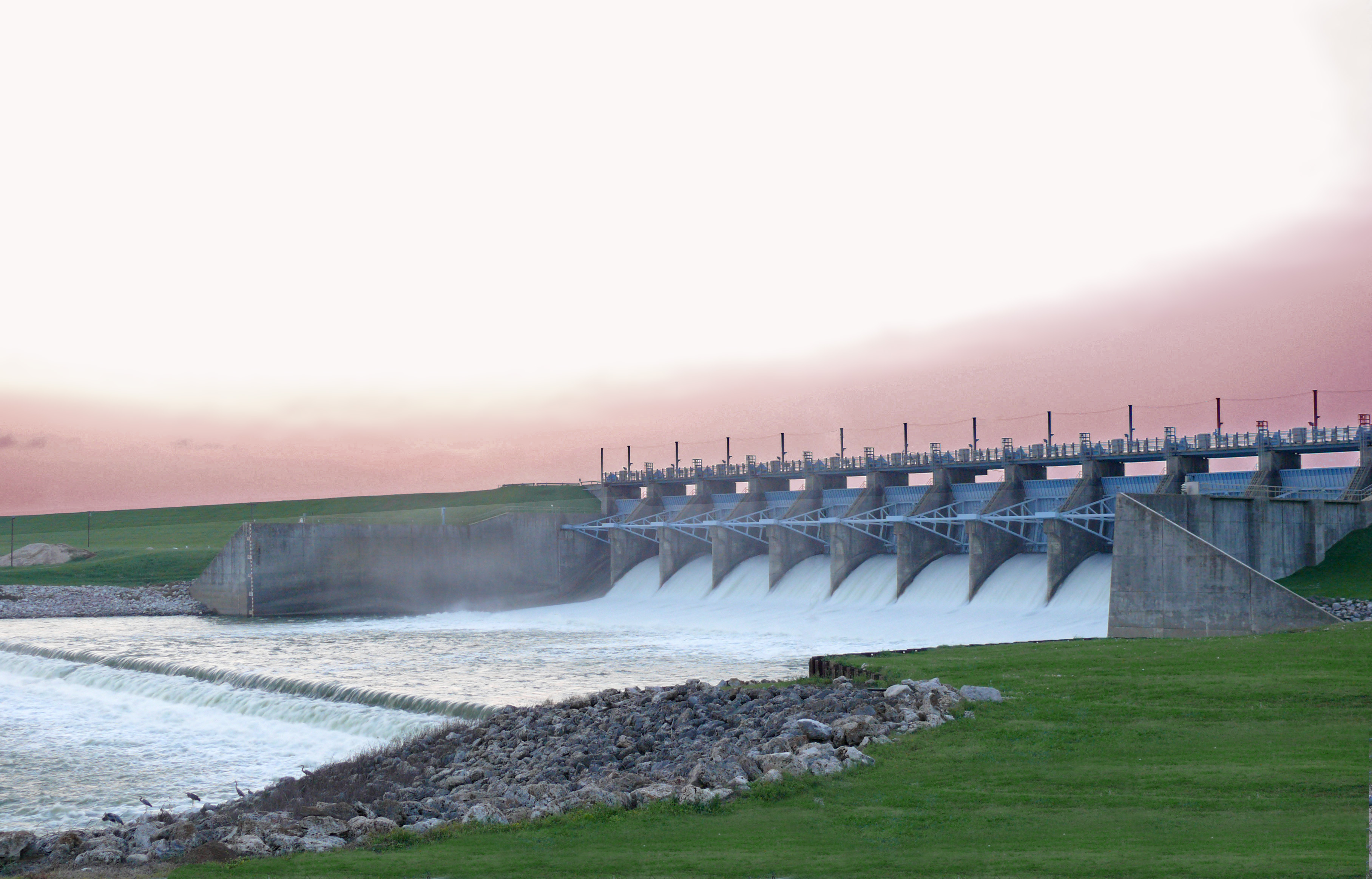
Livingston Dam
Working first on the effects of Lake Livingston and Livingston dam on sediments of the Trinity River, I confirmed that sediment yields at gaging stations upstream of the lakeand downstream indicate extensive sediment trapping in the lake and a major decline in sediment loads after dam construction. However, the Texas Water Development Board (TWDB) had also collected sediment data further downstream, at the upper end of the fluvial-estuarine transition zone, both before and after dam construction. These data showed NO post-dam reduction and extremely low sediment loads in both periods, suggesting that even before the dam was constructed not much fluvial sediment was making it to the lower river. The TWBD also has a nice database of repeated surveys of small and large lakes throughout the state. Those of the southeast Texas coastal plain showed quite significant sediment produced within the region. I first presented these data in Phillips and Musselman (2003), and in Phillips (2003a), in the context of arguing that long-term sediment yields may change little even when upstream erosion and sediment production changes dramatically. In Phillips et al. (2004) we developed a more detailed sediment budget for the Trinity downstream of the dam. Sediment trapped in the lake is partially offset by channel erosion downstream of the dam (a common phenomenon often referred to as “hungry water”), and by the aforementioned erosion and tributary inputs from coastal plain sources. However, we also found that the lower river is a sediment bottleneck, with storage so extensive that upper and lower basins were essentially decoupled even before the dam. Dam-related sediment starvation effects occur for only 50-60 km downstream.
Though I did not directly examine sediment transport in the Sabine River, found consistent evidence that geomorphic impacts of the large Toledo Bend dam and reservoir were mainly confined to a relatively short zone downstream of the dam, and that effects of sediment trapping and flow regulation were nearly invisible in the lowermost river (Phillips, 2003b; 2008).
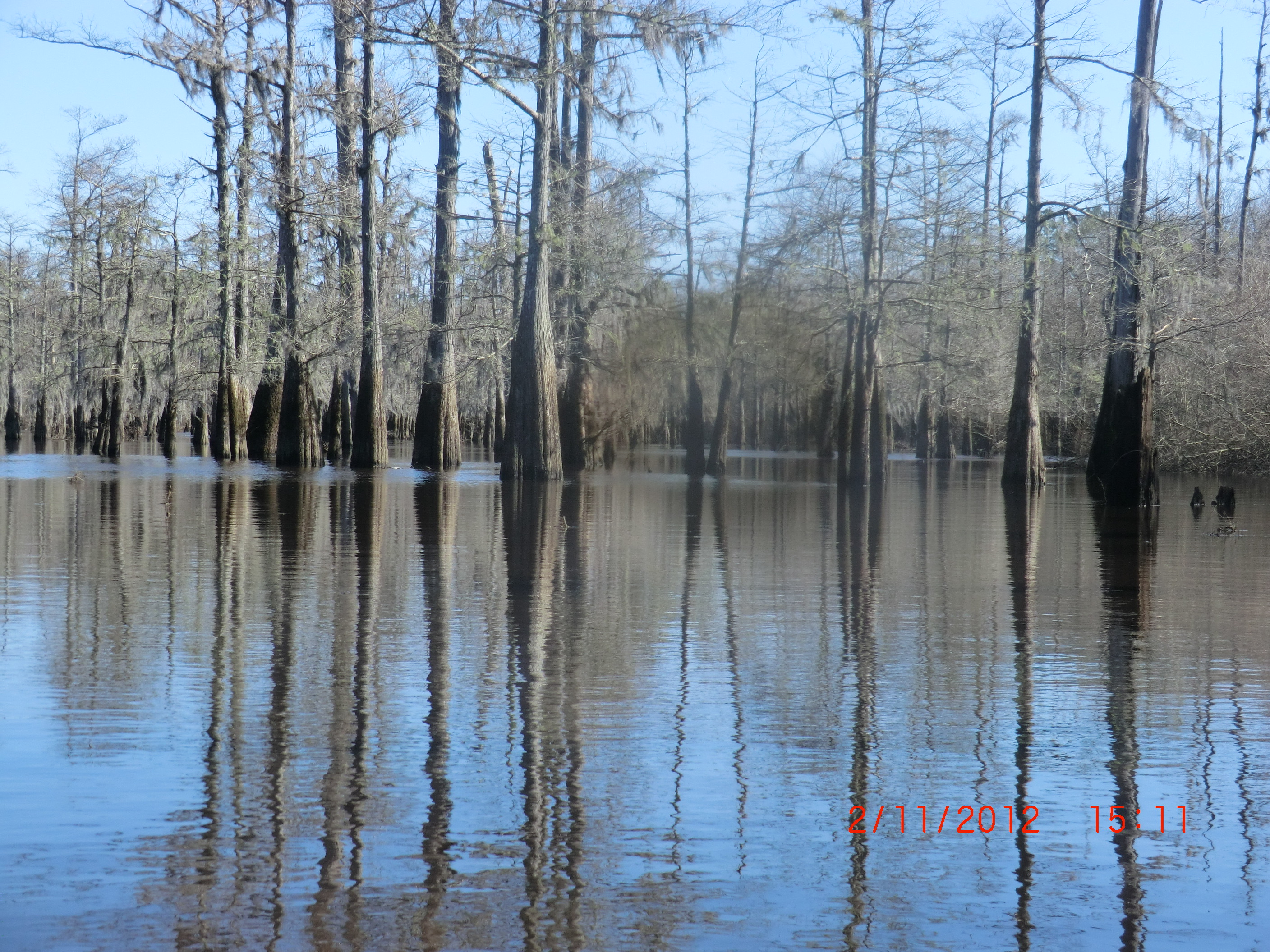
Floodplain depression on the lower Neches River, Texas.
A deeper dive into the lower Trinity showed that the extensive accommodation space in the lower River is strongly linked to antecedent Pleistocene morphology. The latter also results in sediment delivery to floodplain depressions during high flows, and the temporary conversion of tributaries to distributaries. Further, we documented low transport capacity in the lower river, and the absence of expected/presumed downstream trends in discharge, slow, and stream power (Phillips and Slattery, 2007). We also examined sedimentation rates in the Trinity River delta, finding that modern fluvial sediment input is insufficient to account for Holocene sedimentation rates, implying non-fluvial sources in addition to river sediment (Slattery et al., 2010).
Based on our Texas and North Carolina experiences, Mike and I wrote a general overview of sediment storage and sediment delivery to the coast by coastal plain rivers (Phillips and Slattery, 2006; updated in Slattery and Phillips, 2010). A key idea is that in many cases the downstream-most gaging stations on most U.S. coastal plain rivers are a considerable distance from the estuaries, and well upstream of sediment bottlenecks in the fluvial-estuarine transition zones. Thus sediment data from these stations—whether they reflect increased loads from land use change or decreased loads from dams—do not reflect actual transport to the river mouth. We also highlighted the extensive storage bottlenecks in lower river reaches, storage in deltas and estuaries, and coastal backwater effects that extend well upstream of the estuaries.
* * * * *
Back to Rodriguez et al., 2020.
Their results indicate that these coastal depocenters have sediment sources other than fluvial watersheds upstream of dams, and that at least some of these inputs have increased since 1950.
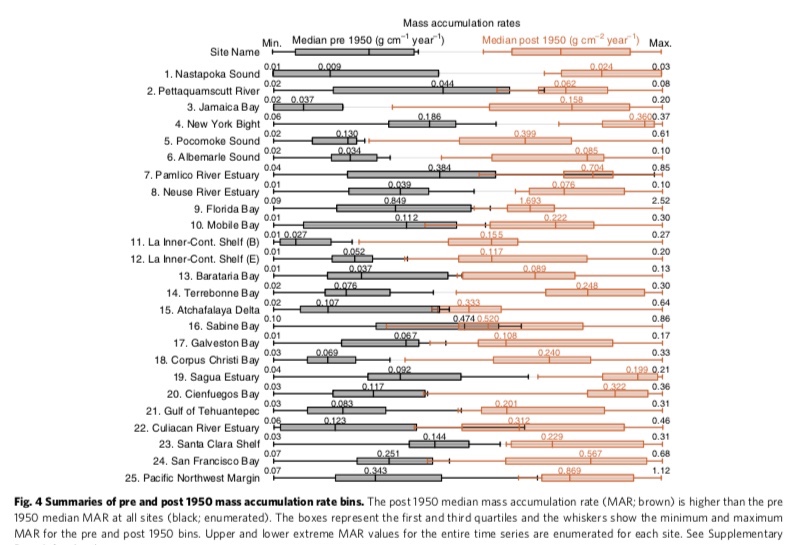
(Rodriguez et al., 2020).
These sediment sources could include onshore transport of marine and coastal sediments and shoreline erosion in estuarine sites (this is, for example, the case in the North Carolina sites, nos. 6-8 in the figure above, and probably others). Longshore transport along ocean and estuarine beaches, and resuspension and redistribution are also sources in some specific locations. Some of the depocenters in the study, such as Sabine Lake and Atchafalaya Bay, have been disturbed by human activities within the estuary, as well.
But Rodriguez et al. attribute the increase mainly to increased erosion and sediment production downstream of dams and lowermost gaging stations, and present evidence of population growth in these areas to support this. I agree—our studies in the North Carolina and Texas coastal plains show plenty of erosion to account for the increases.
* * * * *
Caveats and apologies: Again, the point of this was to highlight these new results and to review (reminisce?) about some of my own related work. Yes, I do feel like these new results support my past work. And yes, I do feel somewhat vindicated with respect to what we were finding in the 1990s and early 2000s. But I do not claim that we were the only ones discovering similar things, or that our work was not both inspired and informed by the studies of others.
References
Phillips, J.D. 1991a. Fluvial sediment budgets in the North Carolina Piedmont. Geomorphology 4: 231-241.
Phillips, J.D. 1991b. Fluvial sediment delivery to a Coastal Plain estuary in the Atlantic Drainage of the United States. Marine Geology 98: 121-134.
Phillips, J.D. 1991c. Upstream pollution sources and coastal water quality protection in North Carolina. Coastal Management 19: 439-449.
Phillips, J.D. 1992a. Delivery of upper-basin sediment to the lower Neuse River, North Carolina, U.S.A. Earth Surface Processes and Landforms 17: 699-709.
Phillips, J.D. 1992b. The source of alluvium in large rivers of the lower Coastal Plain of North Carolina. Catena 19: 59-75.
Phillips, J.D. 1993. Pre- and post-colonial sediment sources and storage in the lower Neuse River basin, North Carolina. Physical Geography 14: 272-284.
Phillips, J.D. 1997. A short history of a flat place: Three centuries of geomorphic change in the Croatan. Annals of the Association of American Geographers 87: 197-216.
Phillips, J.D. 2003a. Alluvial storage and the long term stability of sediment yields. Basin Research 15: 153-163.
Phillips, J.D. 2003b. Toledo Bend Reservoir and geomorphic response in the lower Sabine River. River Research and Applications 19: 137-159.
Phillips, J.D. 2008. Geomorphic controls and transition zones in the lower Sabine River. Hydrological Processes 22: 2424-2437.
Phillips, J.D., Golden, H., et al. 1999. Soil redistribution and pedologic transformations on coastal plain croplands. Earth Surface Processes and Landforms 24: 23-39.
Phillips, J.D., Musselman, Z.A. 2003. The effect of dams on fluvial sediment delivery to the Texas coast. Coastal Sediments ‘03. Proceedings of the 5th International Symposium on Coastal Engineering and Science of Coastal Sediment Processes, Clearwater Beach, Florida, p. 1-14.
Phillips, J.D., Slattery, M.C. 2006. Sediment storage, sea level, and sediment delivery to the ocean by coastal plain rivers. Progress in Physical Geography 30: 513-530.
Phillips, J.D., Slattery, M.C., 2007. Downstream trends in discharge, slope, and stream power in a coastal plain river. Journal of Hydrology 334: 290-303.
Phillips, J.D., Slattery, M.C., Gares, P.A. 1999. Truncation and accretion of soil profiles on coastal plain croplands: Implications for sediment redistribution. Geomorphology 28: 119-140.
Phillips, J.D., Slattery, M.C., Musselman, Z.A. 2004. Dam-to-delta sediment inputs and storage in the lower Trinity River, Texas. Geomorphology 62: 17-34.
Phillips, J.D., Wyrick, M., et al. 1993. Accelerated erosion on the North Carolina Coastal Plain. Physical Geography 14:114-130.
Rodriguez, A.B., McKee, B.A., et al. 2020. Coastal sedimentation across North America doubled in the 20th century despite river dams. Nature Communications 11, 3249; doi.org/10.1038/s41467-020-16994-z |
Slattery, M.C., Gares, P.A. et al. 1998. Quantifying soil erosion and sediment delivery on North Carolina coastal plain croplands. Conservation Voices 1(2): 20-25.
Slattery, M.C., Gares, P.A., Phillips, J.D. 2006. Multiple modes of runoff generation in a North Carolina coastal plain watershed. Hydrological Processes 20: 2953-2969.
Slattery, M.C., Phillips, J.D. 2010. Controls on sediment delivery in coastal plain rivers. Journal of Environmental Management 92: 284-289.
Slattery, M.C., Todd, L.M., et al. 2010. Holocene sediment accretion in the Trinity River delta, Texas, in relation to modern fluvial input. Journal of Soils & Sediments 10: 640-651.
Posted 17 December 2020