History, wrote Tony Horwitz (2008), is an arbitrary collection of facts and observations. Myths are created and perpetuated. To expand a bit in the context of historical Earth and environmental sciences, history is an arbitrary collection of facts and observations, filtered by aspects of historical preservation, and limitations of perception and interpretation. Historical narratives are created, negotiated, and perpetuated. Historical narratives—explanations, chronologies, historical descriptions, chronicles, and, yes, myths—are forms of stories. The key point is that while historical science is (at least at its best) grounded in facts and data, however censored and variably perceived, the reporting and dissemination thereof is in the form of created, negotiated, and perpetuated stories.
The vast majority of historical narratives in Earth and environmental sciences and interpretations of observed temporal patterns, I argue here, can be characterized in terms of nine basic storylines:
1. Steady-state equilibrium. These are balance-of-nature narratives, indicating that landscapes proceed toward some state of stability or balance. Equilibrium theory in geosciences, pedology, and ecology underpins this storyline.
2. Progression to climax. These plots are often, but not always, tied to equilibrium notions in that the climax states are often assumed to be stable steady states. This storyline is based on progression toward some predetermined end-point state, though the possibility of disruption or disturbance is often part of the story. Classical succession theory in ecology, progressive development toward mature zonal soils in pedology, and the Davisian cycle of erosion in geomorphology are examples of this storyline.
3. Progress, progressive development, improvement. Biological evolution and landscape development are portrayed as involving a general improvement over time—e.g., organisms become better adapted, ecosystems optimize mass and energy fluxes, flow networks increase in efficiency, etc. These storylines can be consistent with equilibrium, climax, and preordained path (see below) plots, but also with stories based on selection.
4. Inevitable, preordained path to present. These narratives occur in at least two different varieties. In some, particularly pre-late-20th century science and natural history, the present is implicitly or explicitly viewed as the preordained conclusion of historical development. Historical narratives are thus geared toward explaining how the current situation came to be. The second variety does not view the present as preordained in any teleological or normative sense, but rather is based on the idea that given the sequence of events on the planet, the present condition was the only possible outcome.
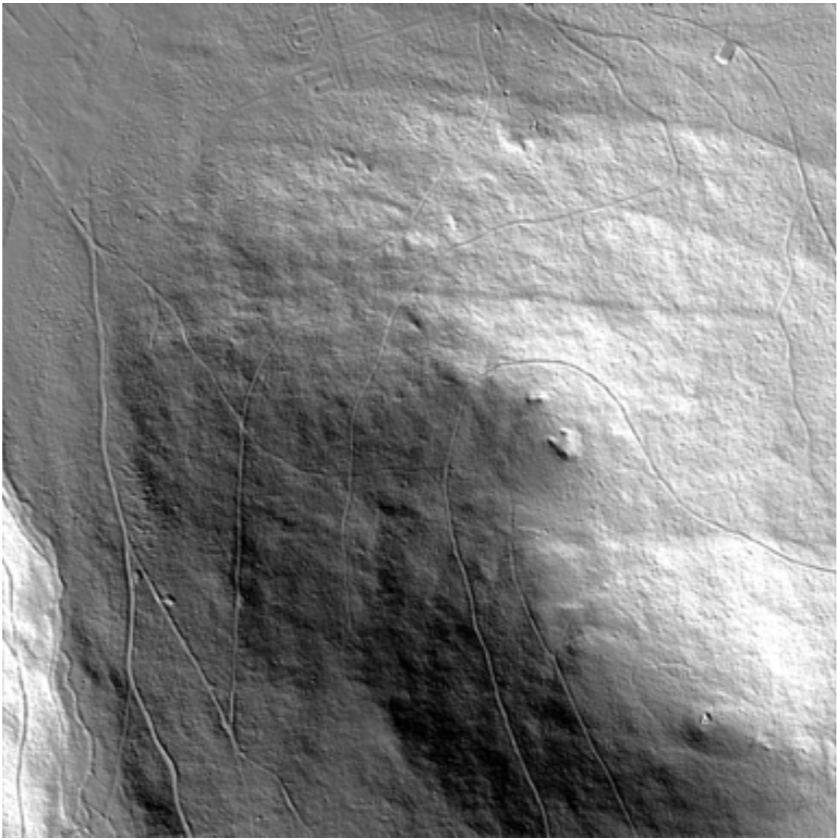
Detailed relief map of a section of the Sumava Mountains (near Polednik), Czech Republic, based on high resolution LiDAR data (courtesy of Sumava National Park, CZ). Note the lack of fluvial dissection. I am currently involved in research seeking to explain how/why, in this moist climate, few channels have formed at the higher elevations and on the mountain slopes. While this may be eventually interpreted in terms of other storylines, at the moment it is a "how did the current condition come to be" story.
5. Chaos, chance, and nonequilibrium. This type of storyline often emphasizes the role of chaotic dynamics and dynamical instabilities in opening up multiple possibilities for both evolutionary pathways and potential outcomes. However, these plots are not necessarily linked to complex nonlinear dynamics; they may simply recognize the role of chance, randomness, and path dependence.
6. State change storylines narrate historical development as a series of changes in landscape conditions or states. They are consistent with, but often not explicitly linked to, state-and-transition models and concepts. While single-path, single-outcome trajectories can be accommodated by state-and-transition frameworks, these narratives are generally used to emphasize multiple-path, multiple-outcome histories, potential reversibility of development, and contingency.
7. Selection. Historical narratives with selection plots recognize the possibility of multiple pathways and potential roles for chance but emphasize one or more overriding selection principles that constrain evolution. Generally, these are based on probabilistic rather than deterministic notions—that is, the selected-for attributes are more likely, but not inevitable or predetermined.
Figure 7 from Phillips (2019) on evolutionary pathways in soil development, based on studies of the North Carolina coastal plain. This story has elements of the chaos/nonequilibrium, state change, and selection storylines (see source article).
8. Self-organization storylines explain landscape evolution in terms of a tendency toward some form of autoregulation, via autocatalysis rather than (or at least independently of) external forcings and boundary conditions. Self-organization plots may be consistent with other storylines, especially equilibrium and selection. They may involve goal functions, whereby landscapes are said to maximize, minimize, or optimize some factor (in which case there is overlap with progressive development/improvement storylines). Self-organization plots may also be based on a predetermined state, such as in theories of self-organized criticality.
9. Unsolved mystery. Some storylines identify phenomena that either cannot be explained by existing hypotheses or theories, or for which evidence is insufficient to apply one of the other storylines. These narratives typically emphasize landscapes that are considered anomalous or oddities in some way, or for which there are multiple competing explanations with no consensus. Classic examples include the origins of the Carolina Bays of the southeastern U.S.A. (Zamora, 2017), the “sliding rocks” of racetrack playa in Death Valley, U.S.A. (Hooke and Jones, 2015), the “fairy circles” of Namibia (Tschinkel, 2015), and the origin of life on Earth.
These storylines relate directly or indirectly to the outcomes of landscape evolution, and are thus related to the idea of attractors, goal functions, and other purported end-points or governing factors. That will be the subject of the next post.
---------------------------------------------
Hooke, R.LeB., Jones, R. 2015. Racetrack Playa: Rocks moved by wind alone. Aeolian Research 19A, 1-3.
Horwitz, T. 2008. A Voyage Long and Strange: Rediscovering the New World. New York: Henry Holt.
Phillips, J.D., 2019. Evolutionary pathways in soil-geomorphic systems. Soil Science 184: 1-12 (attached).
Tschinkel, W. 2015. Experiments testing the causes of Namibian Fairy Circles. PLoS One 10(10): e0140099. doi: 10.1371/journal.pone.0140099
Zamora, A. 2017. A model for the geomorphology of the Carolina Bays. Geomorphology, 282, 209–216. https://doi.org/10.1016/j.geomorph.2017.01.019
Posted 2 December 2020
Comments or questions? Contact me at jdp@uky.edu.